Spotlight on Research: Charting the Future Through Bioengineering
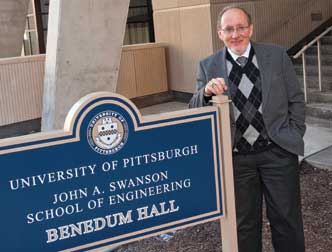
The science of bioengineering is profoundly changing the world of medicine as we know it. Continued research and technological breakthroughs have revolutionized the discipline, resulting in advances never before thought possible: a tiny cardiac-assist device for infants whose hearts don’t pump adequately, a blood-treatment tool that allows ventilator-dependent people to breathe without a ventilator, a high-tech bandage used successfully to repair injured hearts, and materials that help regenerate bone and then dissolve once the job is done.
University of Pittsburgh researchers are at the forefront of this surge in innovation. They are applying their expertise to create a myriad of new devices, spurring the creation of start-up companies as well as research partnerships involving an array of industries.
Such accomplishments are in no small part the result of the idea-nurturing environment that researchers find on the University’s Pittsburgh campus and across Western Pennsylvania. In a field that demands collaboration, Pitt researchers find a wealth of leading experts in diverse disciplines within the University’s Swanson School of Engineering, its School of Medicine, and its other schools of the health sciences; the University of Pittsburgh Medical Center (UPMC) health network; and the Pitt-UPMC McGowan Institute for Regenerative Medicine.
The results have been widely recognized, with Pitt in recent years being ranked as one of the nation’s top universities for bioengineering research and education. In 2010, for example, U.S. News and World Report ranked Pitt’s graduate program in bioengineering 12th in the nation overall, along with the University of Michigan and University of California, Berkeley. Among public institutions, Pitt was ranked fourth best. In the 2010 rankings by the National Research Council, the most comprehensive, data-based assessment of research-doctorate programs in the United States, Pitt’s bioengineering program was ranked seventh out of 53 programs.
Another indicator of the caliber of Pitt’s bioengineering research is the amount of funding the Swanson School’s Department of Bioengineering receives from competitive federal funding programs. In 2009 and 2010 alone, Pitt bioengineering researchers were awarded $5.6 million to develop a heart-assist pump for infants and toddlers, part of a $23.6 million effort by the National Institutes of Health’s (NIH) National Heart, Lung, and Blood Institute, and $5.1 million from the NIH to explore new methods for growing cells from existing tissues and organs. In addition, Pitt was selected as one of the leaders of a national $85 million program to advance regenerative medicine and develop treatments for wounded soldiers. Funding for that program stems from a new federal entity, the Armed Forces Institute of Regenerative Medicine.
“We are a national program,” says Pitt’s Harvey Borovetz, Distinguished Professor, chair of the bioengineering department, Robert L. Hardesty Professor of Surgery, and a professor of chemical and petroleum engineering. “And we’re a national program because we have unique strengths and collaborations.”
Bioengineering as a discipline experienced significant growth during the final decades of the 20th century. Its rise in prominence was fueled by funding from the federal government and private benefactors, primarily The Whitaker Foundation, whose dollars helped establish many of the bioengineering departments found on university campuses today, including Pitt’s.
Pitt’s Department of Bioengineering was established in the Swanson School of Engineering in 1998. Today it includes 23 full-time faculty, more than 100 faculty holding secondary appointments in bioengineering, 180 undergraduate students, and about 180 graduate students, two-thirds of whom are PhD candidates.
The collaborative nature of Pitt’s bioengineering environment is a key factor in the department’s ability to enroll a significant number of PhD candidates, half of whom are mentored by Pitt School of Medicine faculty.
Robust Collaboration
“At the end of the day, what NIH generally chooses to fund is innovative, significant work that will benefit public health,” says Borovetz. “And for many bioengineers, it’s hard to imagine working on innovative, significant work that benefits public health and not working with colleagues who are public-health practitioners.”
Bioengineering collaborations with the School of Medicine provide clinical insight into just about every medical specialty. Such partnerships allow bioengineers to identify which clinical issues they may help resolve. In addition, UPMC offers a broad network of 20 hospitals and renowned programs in such specialties as transplantation, cancer, neurosurgery, cardiology/cardiac surgery, orthopaedics, and sports medicine.
A case in point: the development of the PediaFlow™ ventricular-assist device for children and newborns with congenital and/or acquired heart disease. Cardiac experts within the medical school were essential during the early research stages for the device, and they continue to spearhead the device’s development as it moves toward clinical trials, Borovetz says. He and a team of experts—who include pediatric heart surgeons and cardiologists from Children’s Hospital of Pittsburgh of UPMC, bioengineering faculty at Pitt and Carnegie Mellon University, and industry partners—are working to refine the device.
And the PediaFlow™ effort is more than an academic exercise. Eight out of every 1,000 infants are born with heart defects, according to a recent report from the American Heart Association. “In many cases, there are few options for these children,” says Borovetz.
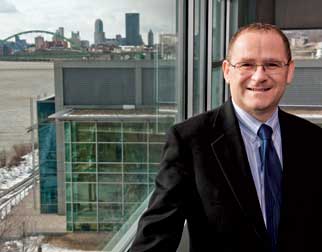
Another uncommon resource available to Pitt researchers is the McGowan Institute for Regenerative Medicine, a Pitt-UPMC entity created in 2001 to better position Pitt and the region in the burgeoning regenerative medicine industry. Based on Pittsburgh’s South Side, the McGowan Institute is an outgrowth of the McGowan Center for Artificial Organ Development, which was established in 1992 with an initial focus on cardiopulmonary organ replacements.
Today, millions of sponsored research dollars flow through Pitt annually to support work related to regenerative medicine. More than 240 faculty are affiliated with the McGowan Institute; their core competencies are broad—cellular biology, gene therapy, imaging, biomaterials, bioengineering, and biomechanics among them—and they use their expertise to develop medical devices, biomaterials, engineered tissue, and cell-based therapies affecting everything from the heart and vascular tissue to the lungs and nervous system.
Not only does such breadth of expertise provide for rich collaborations within the University, it also attracts privately funded entities, enticing them to form the partnerships that the McGowan Institute views as part of its mission. “We are seen as a place where people do serious work in regenerative medicine. And, to some degree, we are a magnet to companies that are interested in that space,” says Alan Russell, director of the McGowan Institute and University Professor of Surgery in Pitt’s School of Medicine.
Several companies have also evolved from the work of Pitt bioengineering researchers. Russell’s work in the decontamination of chemical weapons through the use of enzymes led to the creation of Agentase, a South Side company that is now a subsidiary of ICX Technologies. Agentase develops products for detecting and addressing chemical contamination in settings that range from war zones to hospitals.
More recently, Russell’s lab is focusing on deciphering the complex chemistry that would allow researchers to more effectively and efficiently separate cells as part of their work to develop new regenerative medicine technologies. “Surfaces that interact with certain cells in interesting ways is of significant interest to us,” says Russell. “In regenerative medicine, people are separating cells all of the time. Today, if you want to separate cells you have to modify those cells in some way and use a machine to tease one cell away from another. We are interested in whether it is possible to design surfaces that wouldn’t require you to modify the cell. Then you could begin to classify and separate cells on the basis of their functionality rather than on the basis of what they stick to in a machine.”
Russell’s laboratory is also investigating certain aspects of sugar’s energy-generating capacity in the hopes of developing an alternative power source that would help satisfy the world’s thirst for electricity. “It is definitely feasible,” he says. “Whether it is scalable and how much power you can generate is a different issue. But we can switch on a light bulb with a can of Coke.”
From Lab to Bedside
The field of bioengineering is recognized for researchers’ ability to move new technologies out of the laboratory into practical use. The Sonic Flashlight™, developed by Pitt Professor of Bioengineering George Stetten, is among the many ideas born in Pitt laboratories that have found their way to the marketplace and patients’ bedsides.
The Sonic Flashlight™ is a handheld ultrasound probe with a mirror and miniature display attached to it. The design allows images to be viewed directly by medical practitioners without their having to turn their heads to view a screen, as is required in traditional ultrasound imaging. The probe enables users to see what lies beneath the skin as a real-time, floating image.
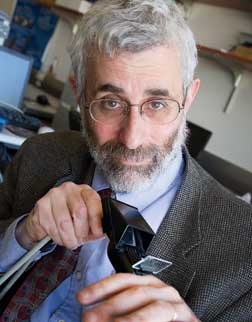
UPMC clinical trials demonstrated the value of the device in helping nurses more comfortably and intuitively insert central catheters peripherally into deep veins in patients’ arms. Insituvue, a Pittsburgh-based start-up company, is readying the device for market.
Stetten, however, isn’t finished exploring the idea behind his high-profile invention. “The basic concept of taking an image with a mirror and putting that image right inside the body in real time so that you can aim scalpels at it doesn’t just have to involve ultrasound,” he says.
He is now developing guidance systems for eye surgery in collaboration with Joel Schuman, chair of Pitt’s Department of Ophthalmology and a professor of bioengineering. The new device, known as an in-situ guidance system, uses optical coherence tomography (OCT) instead of ultrasound; OCT is an imaging modality that employs light waves to produce high-resolution images. It’s like ultrasound, but with a laser, and it can peer into the cornea and the retina, where it displays high-resolution 3-D images of what is there.
Traditionally, OCT hasn’t been used during eye surgery because the surgeon would have to turn away from the microscope to view images projected on a screen. Stetten’s device, much like the Sonic Flashlight™, displays the real-time images inside the eye, directly in the surgeon’s field of vision through the microscope, as if he or she is wearing X-ray glasses.
Studies of the psychophysics of performing such delicate operations, done in collaboration with experts at Carnegie Mellon University, found, not surprisingly, that it is much better for surgeons to be able to aim a scalpel or needle at a target they see in front of them than to have to look away at a screen. “It’s more accurate and easier to learn, and it’s generally more comfortable to be interacting with in-situ images,” says Stetten. “To be able to do microsurgery on the eye having a real-time OCT image right where the tip of your needle is is a big advantage.”
Innovative Therapies for Lung Disease and Sepsis
People with lung disease are also finding hope in the technologies being developed by Pitt bioengineers, including a device that could make ventilators unnecessary for some patients.
For patients whose lung function is weakened by disease, ventilators remove carbon dioxide from blood and add oxygen so that the body and its organs can function. While such respiratory support is critical, it presents problems of its own, including the risk that the process causes additional damage to already-diseased lungs.
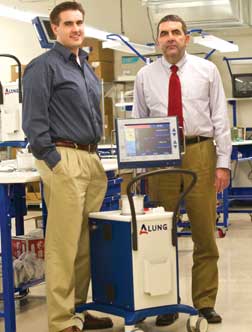
Alung Technologies, a Pittsburgh-based start-up, is conducting clinical trials in Europe involving a respiratory-assist device that does the job of a ventilator—without the ventilator. The product was developed in the laboratory of William Federspiel, the William Kepler Whiteford Professor of Bioengineering, Surgery, and Chemical Engineering.
“It takes a small amount of blood outside the body and runs it through an artificial lung cartridge that was specifically designed to be very efficient at removing carbon dioxide from the bloodstream, and it also adds oxygen into the bloodstream,” says Federspiel.
Federspiel’s laboratory is also exploring the possibility of taking that concept one step further: a device that would perform the same function without having to draw blood from patients. “The idea,” he says, “is to have a catheter that sits in a blood vessel and oxygenates and removes carbon dioxide to provide respiratory support.” This was the dream of one of Federspiel’s early collaborators at Pitt, the late cardiothoracic surgeon Brack Hattler, with whom Federspiel founded Alung Technologies.
Federspiel is also investigating new technologies for treating sepsis, a serious whole-body inflammatory reaction to an infection in blood or tissues. Federspiel’s laboratory is working with John Kellum in the School of Medicine’s Department of Critical Care Medicine to develop a device that filters a sepsis patient’s blood through a cartridge of absorbent beads designed to remove the chemical molecules that orchestrate the body’s innate immune system. “By removing them from the bloodstream, we are able to calm the reaction to this infection and mitigate the effects associated with sepsis,” Federspiel said.
Matters of the Heart
For patients who experience post-ischemic cardiomyopathy, which is heart failure following a heart attack, the prognosis is rarely good. Scar tissue forms, the wall of the heart thins, and the heart’s ability to pump diminishes to the point where extreme measures, such as a transplant, are necessary.
In his laboratory, William Wagner is exploring technologies that could offer such patients a far more attractive option. One is an elastic patch designed to support the damaged part of the heart and help it heal. Another is an injectable gel that at body temperature becomes an elastic girdle to reduce stress on the healing heart tissue.
Both materials are being developed with such tissue-regenerative properties as the ability to deliver drugs that spur healing and the capacity to degrade safely within the body. “We’re developing material that will fulfill a function, often a mechanical function—like an internal crutch or girdle—but will go away over time and allow the body, as it heals, to take over that function on its own,” says Wagner, deputy director of the McGowan Institute and a professor of surgery, bioengineering, and chemical engineering at Pitt.
Many materials used in biomedical applications today were originally made for other purposes, such as for hosiery or wire insulation. But Wagner’s lab designs them from scratch for specific medical situations, aiming for better biocompatibility and function.
For the heart patch, which is surgically implanted over the heart’s damaged area, researchers in Wagner’s lab engineered a thin sheet of polyurethane with several key properties: the elasticity necessary to protect the healing heart tissue’s high stress, the molecular makeup to degrade into materials that are not toxic to the body, and the ability to precisely deliver “growth factors,” which are naturally produced proteins that stimulate cell division and promote tissue regeneration.
In addition, Wagner can tweak the material’s molecular design to make it softer or stiffer, degrade faster or slower, break down with a specific enzyme, and deliver a specific drug.
Wagner’s team is also exploring a thermoresponsive hydrogel engineered to be a liquid at cold temperatures that quickly forms an elastic patch at body temperature. Such properties make it possible to administer support to a damaged heart by way of injection rather than through open-chest surgery.
“The question we’re addressing with all of this is whether there is something we can do to improve the outcomes of these patients before the heart completely fails,” says Wagner.
Devices as Living Tissue
In the laboratory of Michael Sacks, the John A. Swanson Endowed Chair in the Department of Bioengineering, advances are being made in understanding the complex structure and function of human heart valves. One long-term goal is for young children with congenital defects to no longer have to endure multiple valve surgeries as they reach maturity. Surgical replacement of the pulmonary heart valve in young children is often initially very successful, yet problems occur as these children literally outgrow the implanted valve. “Pediatric cardiac surgeons have to go in every several years to replace it with another valve,” says Sacks. “It often takes multiple surgeries to bring that child to adulthood. One of the big challenges is that all of those surgeries have associated mortality and morbidity.”
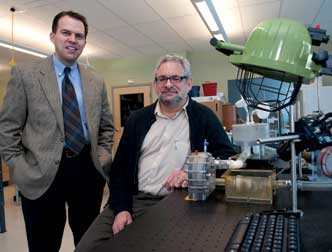
Sacks, one of the world’s leading authorities in cardiovascular biomechanics, is applying his expertise to gaining deeper insight into the heart valves’ structure and function—a critical step toward engineering a living replacement valve that will grow with the child, just like a healthy natural valve would.
To create a living-tissue replacement heart valve, researchers are investigating a strategy that involves seeding a biocompatible polymer scaffold with cells. The cells, once implanted, would grow a living-tissue valve capable of functioning and developing as a natural heart valve does. The research is a collaborative effort between Sacks’ laboratory, a research colleague’s laboratory at Children’s Hospital Boston, and William Wagner’s laboratory at Pitt.
Work on such a replacement valve for children is still in the early stages. One major challenge is to understand what happens when the replacement valve is implanted in a living heart and the body begins the process of remodeling.
“What we have been able to do is gain a deeper understanding of how the native valve works,” says Sacks. “In terms of the valve, we’ve made a lot of strides in understanding how to fabricate the scaffolds, how to model them, and how to seed and grow valve-like tissues. While clinical trials remain a long-term goal, we currently have a much better understanding of what our design endpoints are, how the valve works, and what is necessary to replace it.”
Restoring Neural Function
Brain damage from injury or disease can often result in the permanent loss of function, such as the ability to move an arm. But Pitt bioengineers are developing technologies aimed at helping patients regain such abilities and improve their quality of life.
In Tracy Cui’s laboratory, researchers are investigating ways to convince the brain to tolerate implantable devices that can, it is hoped, restore some lost function. These researchers are also looking into methods of engineering healthy neural tissue.
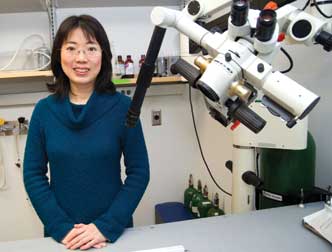
Devices implanted in the brain can be rendered useless if the brain rejects them or covers them with scar tissue. Cui’s laboratory is trying to ensure a better outcome so an electrode that listens to neurons’ electrical signals won’t have those signals muted by scar tissue. The goal is to develop a device that enables patients who’ve lost a certain function to control, for example, a robotic arm with their thoughts.
One strategy for creating a more biocompatible electrode is to coat it with proteins that exist in the brain. “Basically, we try to trick the brain tissue into thinking that the chip is not a foreign body,” says Cui, a Pitt professor of bioengineering. Another approach is to put an electrically switchable polymer on the electrode that, when activated, pumps an anti-inflammatory drug to control scar tissue or neurotrophic factors to promote neuron health. The National Institute of Neurological Disorders and Stroke and the U.S. Department of Defense fund the research.
Cui’s laboratory is also investigating how spinal cord tissue tolerates an implantable device designed to stimulate the neural circuit, restoring arm or leg movement or sensory functions that have been impaired by injury. The underlying concept of the device is similar to that of cochlear implants used to restore hearing loss.
Engineering healthy neural tissue is another approach to restoring brain-directed functions. To grow such tissue, Cui and other Pitt scientists are seeding electrically conductive polymer scaffolds with neural stem cells. The hope is that once the scaffold is implanted, electrical current can be used to coax the stem cells into becoming neurons or other cells necessary to restore lost brain activity.
Soldiers and others who suffer traumatic brain injuries are not the only ones who would benefit, says Cui. “As the population gets older, there’s an increasing number of patients with diseases like Parkinson’s and Alzheimer’s. So we really need to find a way to replace and regenerate neurons.”
Mending Bone
Prashant Kumta is developing advanced technologies to help overcome severe bone defects and injuries. Kumta holds the Edward R. Weidlein Chair in the Swanson School of Enginnering and is a professor in the Departments of Bioengineering, Chemical and Petroleum Engineering, and Mechanical Engineering and Materials Science. Using his expertise in bioceramics and biometallurgy, he is developing implantable materials and delivery systems for ceramics, natural and synthetic polymers, and metals and novel nanoparticles. The goal? To be able to repair “critical bone defects,” such as a missing segment of bone too substantial for the body to heal on its own.
One such advanced material to be tested in clinical trials is a biocompatible and bioresorbable ceramic cement that is injected into the site of the defect as a scaffold. The scaffolds are designed to provide semi-load-bearing support and promote bone regeneration before they safely dissolve. “With these scaffold structures, the cement can be placed at the defect site, and in eight weeks the cement disappears and you have formed new bone,” Kumta says.
The U.S. Department of Defense, which funds the research, is particularly interested in this technology as a way to improve the outcomes of soldiers who have suffered severe craniofacial injuries. Kumta’s collaborators on the project include colleagues in Pitt’s Department of Bioengineering, School of Dental Medicine, and the McGowan Institute.
The cement comprises a calcium phosphate-based ceramic powder and other additives mixed with a fluid that contains nanoparticles on which researchers are able to bind growth factors and other signaling molecules that help regenerate bone.
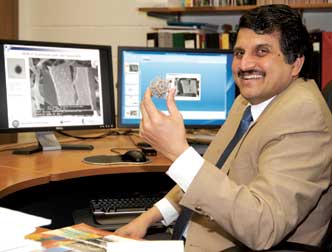
The porous nanostructured cement has the potential to greatly improve the prognosis for those with craniofacial injuries. Kumta is also working toward developing new metal-based technologies to regenerate load-bearing bone, which would enable surgeons to repair defects in the femur—for example. magnesium, in particular, has shown great potential for such applications, since it possesses mechanical characteristics identical to those of natural bone as well as the ability to safely dissolve in the body. Kumta and his colleagues are designing novel magnesium-based alloys to tap those characteristics for the benefit of patients with critical bone defects. “The ultimate dream,” he says, “is that the metal can be placed into a femur, and in a few weeks’ time, when the surgical incision has healed, the patient will be able to stand up and resume normal activities.”
More Work Ahead
Pitt bioengineering researchers like Kumta can look forward to continued demand for the kinds of technologies they have developed.
The aging population, a growing focus on health issues, and other factors are expected to sustain the demand for sophisticated materials, devices, and other technologies that emerge from bioengineering laboratories. “I think bioengineering is in the beginning of its growth phase,” says Russell. “And I think we are a long way off from seeing any change in that.”
Other Stories From This Issue
On the Freedom Road
Follow a group of Pitt students on the Returning to the Roots of Civil Rights bus tour, a nine-day, 2,300-mile journey crisscrossing five states.
Day 1: The Awakening
Day 2: Deep Impressions
Day 3: Music, Montgomery, and More
Day 4: Looking Back, Looking Forward
Day 5: Learning to Remember
Day 6: The Mountaintop
Day 7: Slavery and Beyond
Day 8: Lessons to Bring Home
Day 9: Final Lessons